Sport
George Loxdale 12PL
Fibre types
All skeletal muscles contain a mixture if fibre types. The mix of fibres varies from individual from muscle group to muscle group to muscle group. To a large extent this fibre mix is inherited. However, training can influence the efficiency of these different fibre types. Two main types of striated skeletal muscle can be distinguished on the basis of their speed of contraction: type 1 (slow twitch) and type 2 (fast twitch).
Characteristics of type 1 muscle fibres
Type 1 (slow-twitch) fibres contract slowly with less force. They are slow to fatigue and suited to longer duration aerobic activities. They have a rich blood supply and contain many mitochondria to sustain aerobic metabolism. Type 1 fibres have a high capacity for aerobic respiration. They are recruited for lower-intensity, longer-duration activities such as long distance running and swimming. A sporting example of type 1 muscle fibres would be long distance running for example marathons.
Characteristics of type 2a fibres
Type 2a fibres (also called fast-twitch or fast-oxidative fibres) are fast-contracting and able to produce a great force, but are also resistant to fatigue. These fibres are suited to middle-distance events.
A sporting example of type 2a fibres would be running at not a full intensity for example running an 800m race.
Characteristics of type 2b muscle fibres
Type 2b fibres (also called fast-twitch or fast-glycolytic fibres) contract rapidly and have the capacity to produce large amounts of force, but they fatigue more readily, making them better suited to anaerobic activity. They depend almost entirely on anaerobic metabolism and are recruited for higher-intensity, shorter-duration activities. They are important in sports that include many stop-go or change-of-pace activities. A sporting example of type 2b muscle fibres is sprinting for example running a 100m race.
All types of muscle fibre are used in all types of activity. Although type 1 fibres are particularly adapted to low-intensity aerobic endurance work, they are generally employed at the beginning of exercise, regardless of the intensity of effort. Type 2 fibres adapt to high-intensity anaerobic exercise involving explosive or powerful movements, but are increasingly employed as fatigue sets in during low-intensity endurance work.
Energy systems
All movement requires energy. The methods by which the body generates energy are determined by the intensity and duration of the activity being undertaken. Activities that require short bursts of effort, such as sprinting or jumping, require the body to produce large amounts of energy over a short period, whereas activities like marathon running or endurance cycling require continued energy production over a longer period and at a slower rate. It is the energy systems of your body that facilitate these processes.
Energy is required in order to make the muscle fibres contract. This energy is obtained from the oxidation of foods in the diet, particularly carbohydrate and fat. When these substances are burned in the muscle cells, ATP is formed, which is rich in energy. When ATP is broken down, it gives energy for muscle contraction. It is the only molecule that can supply the energy used in the contraction of muscle fibres and can be made in three ways: the creatine phosphate system which is also called Phosphocreatine, the lactic acid energy system and the aerobic energy system.
Creatine phosphate energy system
ATP and creatine phosphate (or phosphocreatine, or PCr) make up the ATP-PCr system. it is the immediate energy system. Creatine phosphate (PCr) is a high-energy compound. When evercise intensity is high, or energy needs are instantaneous, creatine phosphate stored in muscle is broken down to provide energy to make ATP. When the high-energy bond in PCr is broken, the energy it releases is used to resynthesise ATP.
In this process, ATP is usually made without the presence of oxygen. Explosive work can be achieved, but only for short periods (up to about 10 seconds) at maximum intensity, as the supply of PCr is very limited.
Lactic acid energy system
This is the short-term energy system. To meet energy requirements for higher intensity over a long period, such as during a 400-metre race, ATP can be made by the partial breakdown of glucose and glycogen. This is an anaerobic process (it does not include oxygen) and therefore is not sustainable over a long duration. Around 60 to 90 seconds of maximal work is possible using this system.
Anaerobic glycolysis
When the ATP-PCr system begins to fade at around 10 seconds, the process of anaerobic glycolysis begins to occur. This system breaks down liver and muscle glycogen stores without the presence of oxygen, which produces lactic acid as a by-product. This limits energy production via this process.
Lactic acid production
Lactic acid is the limiting factor of the anaerobic system. it accumulates and diffuses into the tissue fluid and blood. if this substance is not removed by the circulatory system, it builds up to impede muscle contraction and cause fatigue. You may have experienced this during intense exercise as an uncomfortable burning sensation in your muscles.
Creatine phosphate system:
ADP + creatine phosphate --> ATP + creatine
Lactic Acid Energy System:
Glucose --> 2ATP + 2 lactic acid + heat
Glycogen --> 3 ATP + 2 lactic acid + heat
Aerobic energy system:
Glucose + oxygen -->
38 ATP + carbon dioxide + water + heat
Fatty acids + oxygen -->
129 ATP + carbon dioxide + water + heat
Aerobic Energy system
This is the long-term energy system. if plenty of oxygen is available, as it is during everday movements and light exercise, glycogen and fatty acids break down to yield large amounts of ATP. This produces carbon dioxide and water, which do not affect the muscles' ability to contract.
Aerobic energy production occurs in the mitochrondia (mitochrondia - organelles enzymes responsible for energy production. Mitochrondria are therefore the part of a muscle responsible for aerobix energy production.) of the cells. these are the power stations of the cells, responsible for converting the food ingested by the cells into energy. the production of energy within the aerobic system is slow to engage because it takes a few minutes for the heart to deliver oxygenated blood to working muscles. Long, continuous and moderate exercise produces energy using this system.
Energy continuum
the body's ability to extract energy from food and transfer it to the contractile proteins in the muscles determines your capacity to exercise for different durations at differing intensities. Thousands of complex chemical reactions are responsible for this energy transfer. the body maintains a continuous supply of energy through the use of adenosine triphosphate (ATP), which is often referred to as the energy currency of the body.
ATP consists of a base (adenine) and three phosphate groups. it is formed by a reaction between an adenosine diphosphate (ADP) molecule and a phosphate. ATP is a versatile molecule that can be used for many things. Energy is stored in the chemical bonds in the molecules. When a bond is broken, energy is released. When a bond is made, energy is stored. When ADP binds another phosphate, energy is stored that can be used later. when a molecule of ATP is combined with water, the last group splits off and energy is released.
The energy systems of the body can function aerobically (with oxygen) or anaerobically (without oxygen.) Movements that require sudden bursts of effort are powered by anaerobic systems, whereas prolonged activities are aerobic.
Energy requirements of different sport and exercise activities
all three energy systems are active at any given time, but depending on the intensity and duration of activity undertaken, different energy systems will be the primary energy provider. heres what happens when you start running:
- the muscle cells burn off the ATP they already contain in about 3 seconds/
- the creatine phosphate system kicks in and supplies energy for 8-10 seconds. this would be the major energy system used by the muscles of a 100- metre sprinter or a weightlifter, where rapid acceleration, short-duration exercise occurs
- if exercise continues longer, the lactic acid energy system kicks in. this would be true for short-distance exercises such as a 200- or 400- metre run or a 100-metre swim.
- if exercise continues, the aerobic energy system takes over. this would occur in endurance events such as an 800-metre run, a marathon run, rowing, cross-country skiing and distance skating
Energy Systems Used in Sports
The three energy systems do not work independently of one another. From very short, very intense exercise, to very light, prolonged activity, all three energy systems make a contribution however, one or two will usually predominate (5).Two factors of any activity carried out affect energy systems more than any other variable they are the intensity and duration of exercise. Here is a list of sports and approximately how the each of the energy systems contributes to meet the physical demands:
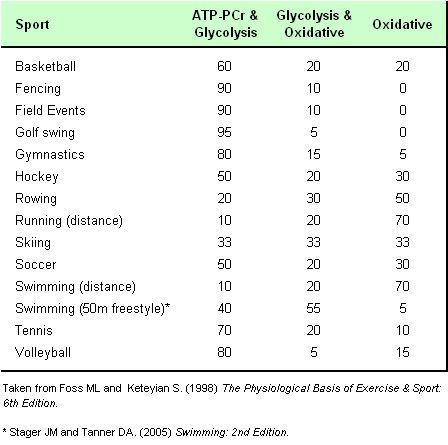
Function of the respiratory system
Gaseous exchange
Gaseous exchange occurs by diffusion between air in the alveoli and blood in the capillaries surrounding their walls. The concept of partial pressure applies the diffusion of gases from a gas mixture to a gas in solution and vice versa. Gases in contact with a liquid dissolve into solution by diffusion until equilibrium is achieved. At equilibrium, the partial pressure of the gases is the same in both gaseous and liquid states, and the gases are diffusing in and out of each state at the same rate. blood entering the capillaries from the pulmonary arteries has a lower oxygen content and a higher carbon dioxide content than the air in the alveoli. oxygen diffuses into the blood via the surface of the alveoli, through the thin walls of the capillaries, through the red blood cell membrane and finally latches on to haemoglobin. carbon dioxide diffuses in the opposite direction, the blood into the alveoli.
Mechanisms of breathing
pulmonary ventilation, or breathing, is the process by which air is transported into and out the lungs, and it can be considered to have two phases. breathing is regulated by the respiratory centres in the brain and stretch receptors within the air passages and lungs. it requires the thorax to increase in size to allow air to be taken in, followed by a decrease to allow air to be forced out.
Inspiration
with inspiration the intercostal muscles contract to lift the ribs upwards and outwards, while the diaphragm is forced downwards and the sternum forwards. this expansion of the thorax in all directions causes a drop in pressure below that of atmospheric pressure, which encourages air to flood into the lung. at this point, oxygen is exchanged for carbon dioxide through the capillary walls.
Expiration
Expiration follows inspiration as the intercostal muscles relax, the diaphragm extends upwards and the ribs and sternum collapse. at that point, pressure within the lungs is increased the air is expelled. when the body is in action, greater amounts of oxygen are required, requiring the intercostal muscles and diaphragm to work harder.
Lung volumes
Your respiratory rate is the amount of air you breathe in one minute. for a typical 18-year old, this represents about 12 breaths per minute at rest, during which time about 6 litres of air passes through the lungs. it can increase significantly during exercise, by as much as 30 to 40 breaths per minute.
Tidal volume
tidal volume is the term used to describe the amount of air breathed in and out with each breath. under normal conditions this represents about 500cm3 of air breathed, both inhaled and exhaled. of this, approximately two-thirds (350cm3) reaches the alveoli in the lungs where gaseous exchange takes place. the remaining 150cm3 fills the pharynx, larynx, trachea, bronchi and bronchioles and is known as dead stationary air.
during exercise, tidal volume increases to allow more air to pass through the lungs. the volume of air passing through the lungs each minute is known as the minute volume and is the product of breathing rate and the amount of air taken in with each breath.
the lungs normally contain about 350cm3 of fresh air, 150cm3 of dead air and 2,500 cm3 of air that has already undergone gaseous exchange with the blood.
Inspiratory reserve volume
by breathing in deeply, it is possible to take in more than the usual 350cm3 of fresh air that reaches the alveoli. this is especially important during exercise. in addition to the tidal volume, you can also breathe in up to an additional 3,000cm3 of fresh air. this is known as the inspiratory reserve volume.
Expiratory reserve volume
this can be up to 1,500cm3 and is the amount of additional air that can be breathed out after normal expiration. at the end of a normal breath, the lungs contain the residual volume plus the expiratory reserve volume. if you then exhale as much as possible, only the residual volume remains.

vital capacity
vital capacity is the amount of air that can be forced out of the lungs after maximal inspiration. the volume is around 4,800cm3
Residual volume
the lungs are never fully emptied of air otherwise they would collapse. the air that remains in the lungs after maximal exspiration, when you breathe out as hard as you can, is referred to as residual volume. the volume is around 1,200cm3 for an average male
Total lung capacity
this is your total lung capacity after you have inhaled as deeply and as maximally as you can, after maximal inspiration. it is normally around 6,000 cm3 for an average-sized male.
Control of breathing
neutral control
although breathing seems simple, its control is complex, it involves neurones, cells that conduct nerve impulses, in the reticular formation and pons, both parts of the brain stem. neurones in two areas of the medulla are critical in respiration. these are the dorsal respiratory group (DRG) and the ventral respiratory group (VRG). the VRG is thought to be responsible for the rhythm generation.
Chemical control
other factors that control breathing are the continually changing levels of oxygen and carbon dioxide. sensors responding to such chemical fluctuations are called chemoreceptors. these are found in the medulla and in the aortic arch and carotid arteries.
Respiratory system
The respiratory system is responsible for providing oxygen and removing carbon dioxide, heat and water vapours. all living creatures require oxygen and give off carbon dioxide. oxygen is required for every cell in your body to function.
Structure of the respiratory system
air is usually drawn into your body via the nose, but sometimes via the mouth, and passes through a series of airways to reach the lungs. This series if airways us referred to as the respiratory tract, and can be divided into two main parts. The upper respiratory tract includes the nose, nasal cavity, mouth, pharynx and larynx; and the lower respiratory tract consists of the trachea, bronchi and lungs.

Nasal cavity
your nose is divided into the external nose and the internal nasal cavity. when you breathe in, air enters the cavity by passing through the nostrils. hairs within the cavity filter out dust, pollen and other foreign particles before the air passes into the two passages of the nasal cavity. here the air is warmed and moistened before it passes into the nasopharynx. A sticky mucous layer traps smaller foreign particles, which tiny hairs called cilia transport to the pharynx to be swallowed.
Epiglottis
a small flap of cartilage at the back of the tongue, the epiglottis closes the top of the trachea when you swallow to ensure food and drink pass into your stomach and not your lungs.
Pharynx
the funnel-shaped pharynx connects the nasal cavity and mouth to the larynx (air) and oesophagus (food). commonly called the throat, the pharynx is a small tube that measures approximately 10-13 cm from the base of the skull to the level of the sixth cervical vertebra. the muscular pharynx wall is composed of a skeletal muscle throughtout its length. it is a passageway for food as well as air, so special adaptations are required to prevent choking when food or liquid is swallowed.
Larynx
The larynx or voice box has rigid walls of muscle and cartiliage, contains the vocal cords and connects the pharynx to the trachea. it extends for about 5 cm from the level of the third to sixth vertrebra.
Trachea
the trachea or windpipe denotes the start of the lower respiratory tract. it is about 12cm long by 2cm in diameter. it contains rings of cartilage to prevent it from collapsing and is very flexible. it travels down the neck in front of the oesophagus and branches into the right and left bronchi.
Bronchus
the right and left bronchi are formed by the division of the trachea, they carry air to the lungs. the right bronchus is shorter and wider than the left is a more common site for foreign objects to become lodged. By the time inhaled air reaches the bronchi, it is warm, clear of most impurities and saturated with water vapour. once inside the lungs, each bronchus subdivides into lobar bronchi: three on the right and two on the left. the lobar bronchi: three on the right and two on the left. the lobar bronchi branch into segmental bronchi, which divide again into smaller and smaller bronchi. overall, there are approximately 23 orders of branching bronchial airways in the lungs. because of this branching pattern, the bronchial network within the lungs is often called the bronchial tree.
Bronchioles
Bronchioles are small airways that extend from the bronchi. they are about 1 mm in diameter and are the first airway branches of the respiratory system that do not contain cartilage. bronchioles end in clusters of thin-walled air sacs, known as alveoli.

Lungs
the paired right and left lungs occupy most of the thoracic cavity and extend down to the diaphragm. they hang suspended in the right and left pleural cavities straddling the heart. the left lung is smaller than the right.
Lobes
each lung is divided into lobes; the right lung has three lobes and the left has two.
Pleural membrane and cavity
the lungs are surrounded by membranes known as pleura. these contain a cavity with fluid that lubricates the pleural surfaces as the lungs expand and contract, preventing friction and keeping them airtight.
Thoracic activity
this is the chamber of the chest that is protected by the thoracic wall. it is separated from the abdominal cavity by the diaphragm.
Visceral pleura
the visceral pleura is the innermost of the two pleural membranes. it covers the surface of the lung and dips into the spaces between the lobes.
Pleural fluid
the pleural membranes produce pleural fluid which fills the space between them. this lubricating fluid allows the lungs to glide easily over the thoracic wall during respiration. although the membranes slide easily over each other, their separation is resisted by the surface tension of the pleural fluid that keeps the lung surface in contact with the chest wall.
Alveoli
the bronchioles end in air sacs called alveoli. the 300 million gas-filled alveoli in each lung amount for most of the lung volume and provide an enormous area for gaseous exchange - roughly the size of a tennis court. a dense network of capillaries surrounds the alveoli to facilitate this process. together, the alveolar and capillary walls form the respiratory membrane that has gas on one side and blood flowing past on the other. gasesous exchange occurs readily by simple diffusion across the respiratory membrane. oxygen passes from the alveoli into the blood and carbon dioxide leaves the blood to enter the alveoli.
Diaphragm
the diaphragm separates the chest from the abdomen, it is the most important muscle involved in breathing. contraction of the diaphragm increases the volume of the chest cavity, drawing air into the lungs during respiration, while relaxation involves recoil of the diaphragm and decreases the volume of the chest cavity, pumping out air.
Internal and external intercostal muscles
- the intercostal muscles lie between the ribs. to help with inhalation and exhalation, they extend and contract.
- the internal intercostal musles lie inside the ribcage, they draw the ribs downwards and inwards, decreasing the volume of the chest cavity and forcing air out of the lungs during expiration.

No comments:
Post a Comment